What Is A Cooling Tower?
Most air-conditioning systems and industrial processes generate heat that must be removed and dissipated. Water is commonly used as a heat transfer medium to remove heat from refrigerant condensers or industrial proces heat exchangers. In the past, this was accomplished by drawing a continuous stream of water from a utility water supply or a natural body of water, heating it as it passed through the process, and then discharging the water directly to a sewer or returning it to the body of water. Water purchased from utilities for this purpose has now become prohibitively expensive because of increased water supply and disposal costs. Similarly, cooling water drawn from natural sources Is relatively unavailable because the ecological disturbance caused by the increased temperature of discharge water has become unacceptable.
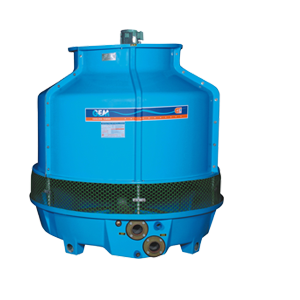
Air-cooled heat exchangers cool water by rejecting heat directly to the atmosphere, but the first cost and fan energy consumption of these devices are high and the plan area required is relatively large. They can economically cool water to within approximately 20°F of the ambient dry-bulb temperature—too high for the cooling water requirements of most refrigeration systems and many industrial processes.
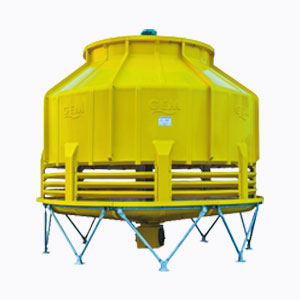
-
Cooling towers overcome most of these problems and therefore are commonly used to dissipate heat from water-cooled refrigeration, air-conditioning, and industrial process systems. The water consumption rate of a cooling tower system is only about 5% of that of a once-through system, making it the least expensive system to operate with purchased water supplies. Additionally, the amount of heated water discharged (blowdown) is very small, so the ecological effect is greatly reduced. Lastly, cooling towers can cool water to within 4 to 5°F of the ambient wet-bulb temperature, or about 35°F lower than can air-cooled systems of reasonable size. This lower temperature improves the efficiency of the overall system, thereby reducing energy use significantly and increasing process output.
A Cooling tower cools water by a combination of heat and mass transfer. Water to be cooled is distributed in the tower by spray nozzles, splash bars, or film-type fill, which exposes a very large water surface area to atmospheric air. Atmospheric air is circulated by (1) fans, (2) convective currents, (3) natural wind currents, or (4) induction effect from sprays. A portion of the water absorbs heat to change from a liquid to a vapor at constant pressure. This heat of vaporization at atmospheric pressure is transferred from the water remaining in the liquid state into the airstream.
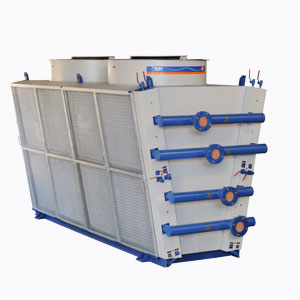
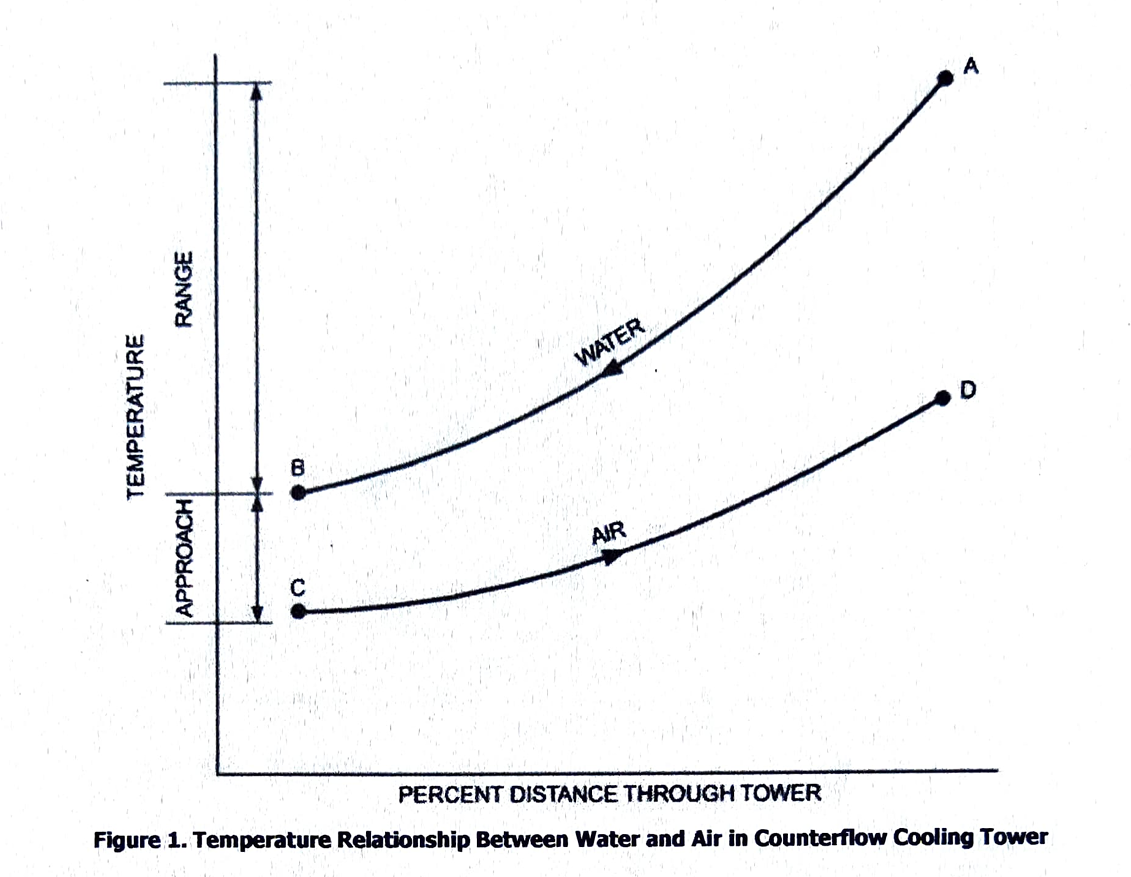
Figure 1 shows the temperature relationship between water and air as they pass through a counterflow cooling tower. The curves indicate the drop in water temperature (A to B) and the rise in the air wet-bulb temperature (C to D) in their respective passages through the tower. The temperature difference between the water entering and leaving the cooling tower (A minus B) is the range. For a steady-state system, the range is the same as the water temperature rise through the load heat exchanger, provided the flow rate through the cooling tower and heat exchanger are the same. Accordingly, the range is determined by the heat load and water flow rate, not by the size or thermal capability of the cooling tower.
The difference between the leaving water temperature and entering air wet-bulb temperature (B minus C) in Figure-1 is the approach to the wet bulb or simply the approach of the cooling tower. The approach is a function of cooling tower capability, and a larger cooling tower produces a closer approach (colder leaving water) for a given heat load, flow rate, and entering air condition. Thus, the amount of heat transferred to the atmosphere by the cooling tower is always equal to the heat load imposed on the tower, whereas the temperature level at which the heat is transferred is determined by the thermal capability of the cooling tower and the entering air wet-bulb temperature.
Thermal performance of a cooling tower depends principally on the entering air wet-bulb temperature. The entering air dry-bulb temperature and relative humidity, taken independently, have an insignificant effect on thermal performance of mechanical-draft cooling towers, but do affect the rate of water evaporation in the cooling tower. A psychrometric analysis of the air passing through a cooling tower illustrates this effect (Figure 2). Air enters at the ambient condition Point A, absorbs heat and mass (moisture) from the water, and exits at Point B in a saturated condition (at very light loads, the discharge air may not be fully saturated). The amount of heat transferred from the water to the air is proportional to the difference in enthalpy of the air between the entering and leaving conditions (h9— hA). Because lines of constant enthalpy correspond almost exactly to lines of constant wet-bulb temperature, the change in enthalpy of the air may be determined by the change in wet-bulb temperature of the air.
Air heating (Vector AB in Figure 2) may be separated into component AC, which represents the sensible portion of the heat absorbed by the air as the water is cooled, and component CB, which represents the latent portion. If the entering air condition is changed to Point D at the same wet-bulb temperature but at a higher dry-bulb temperature, the total heat transfer (Vector DB) remains the same, but the sensible and latent components change dramatically. DE represents sensible cooling of air, while EB represents latent heating as water gives up heat and mass to the air. Thus, for the same water-cooling load, the ratio of latent to sensible heat transfer can vary significantly.
The ratio of latent to sensible heat is important in analysing water usage of a cooling tower. Mass transfer (evaporation) occurs only in the latent portion of heat transfer and is proportional to the change in specific humidity. Because the entering air dry-bulb temperature or relative humidity affects the latent to sensible heat transfer ratio, it also affects the rate of evaporation. In Figure 2, the rate of evaporation in Case AB(WB- WA) is less than in Case DB (WB- WD) because the latent heat transfer (mass transfer) represents a smaller portion of the total.
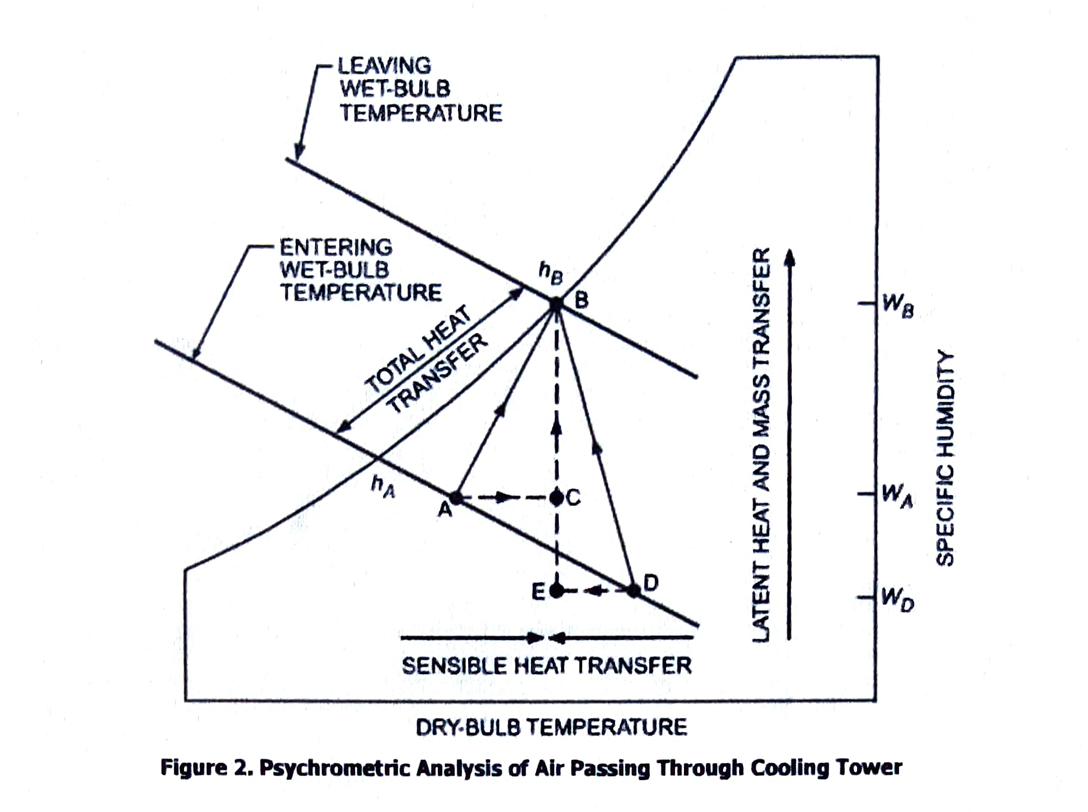
The evaporation rate at typical design conditions is approximately 1% of the water flow rate for each 12.5°F of water temperature range; however, the average evaporation rate over the operating season is less than the design rate because the sensible component of total heat transfer increases as entering air temperature decreases.
In addition to water loss from evaporation, losses also occur because of liquid carryover into the discharge airstream and blowdown to maintain acceptable water quality. Both of these factors are addressed later in this chapter.
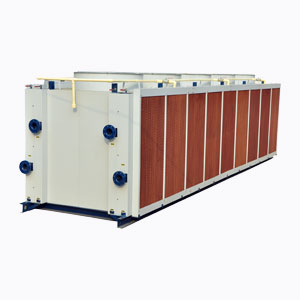
Design Conditions
The thermal capability of any cooling tower may be defined by the following parameters:
- Entering and leaving water temperatures.
- Entering air wet-bulb or entering air wet-bulb and dry-bulb temperatures.
- Water flow rate.
The entering air dry-bulb temperature affects the amount of water evaporated from any evaporative cooling tower. It also affects airflow through hyperbolic towers and directly establishes thermal capability in any indirect-contact cooling tower component operating in a dry mode. Variations in tower performance associated with changes in the remaining parameters are covered in the section on Performance Curves.
The thermal capability of a cooling tower used for air conditioning is often expressed in nominal cooling tower tons. A nominal cooling tower ton is defined as cooling 3 gpm of water from 95°F to 85°F at a 78°F entering air wet-bulb temperature. At these conditions, the cooling tower rejects 15,000 Btu/h per nominal cooling tower ton. The historical derivation of this 15,000 Btu/h cooling tower ton, as compared to the 12,000 Btu/h evaporator ton, is based on the assumption that at typical air-conditioning conditions, for every 12,000 Btu/h of heat picked up in the evaporator, the cooling tower must dissipate an additional 3000 Btu/h of compressor heat. For specific applications, however, nominal tonnage ratings are not used, and the thermal performance capability of the tower is usually expressed as a water flow rate at specific operating temperature conditions (entering water temperature, leaving water temperature, entering air wet-bulb temperature).